RF inductors, known for their versatility, are employed in various construction types to fulfill the specific performance demands of diverse applications. Common applications in RF circuits include matching, resonators, and chokes. Matching is crucial for eliminating impedance mismatches, thus minimizing reflections and losses in lines between circuit blocks like antennas, radio frequency blocks, or intermediate frequency (IF) blocks. In synthesizers and oscillator circuits, resonators are pivotal for tuning the circuit to the desired frequency.
As chokes, inductors are strategically positioned in the power supply lines of functional components, such as RF or IF blocks, primarily to attenuate high-frequency AC currents. The bias tee, a notable component, allows DC current to bias active devices like diodes, combining the DC bias current with the AC/RF signal for output from the AC+DC output port.
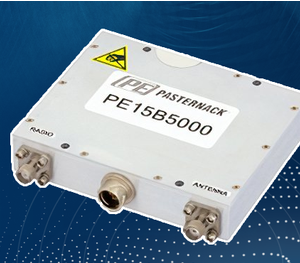
RF Inductor Specifications:
Inductance, a key property of electrical conductors, resists changes in current flow. It's quantified as the ratio of induced voltage to the rate of change in current, measured in Henrys (H). RF inductors typically range from 0.5 nanohenries (nH) to several hundred nanohenries, influenced by factors like construction, core size, material, and coil turns. These inductors are available in both fixed and variable inductance values.
The DC current rating (DCR), related to DC resistance, measures in amperes the current an inductor can handle without overheating or saturating. This metric is significant for assessing an inductor's thermal performance. Higher power loss occurs with increasing current and DC resistance, leading to a rise in inductor temperature. For instance, a component with a 125°C rated ambient temperature experiencing a 15°C rise due to full-rated current (Irms or Idc) would reach a maximum temperature of about 140°C.
Saturation current pertains to the DC current level that diminishes the inductance by a specific value, tied to the core's flux density capacity. This current is integral to the magnetic properties of the inductor, while DCR describes its maximum DC current capacity, linked to its physical characteristics.
Self-resonant frequency (SRF) defines the frequency threshold beyond which the sensor ceases to function. Generally, larger inductance correlates with lower SRF, and vice versa, due to parasitic capacitance effects. Inductors, with low distributed capacitance between terminal electrodes or wound conductor turns, resonate with this capacitance at SRF. At SRF, the inductor behaves as a resistor, and at frequencies above this point, distributed capacitance dominates.
In high-frequency circuit and module selection, considering just the requisite inductance isn't sufficient; the SRF should ideally be over 10 times the operating frequency. For choke applications, the optimal SRF is where impedance peaks, enhancing signal blocking capabilities.
Q-factor, a dimensionless parameter, indicates the underdamping of an oscillator or resonator. It's defined as the ratio of initial energy stored in the resonant cavity to the energy lost per oscillation period. Alternatively, Q-factor is the center frequency to bandwidth ratio of the resonator when driven by oscillation.
High Q values result in narrow bandwidth, critical for LC cell (oscillator) circuit use or narrow bandpass applications. Additionally, a high Q-factor can diminish insertion loss and reduce power consumption. It encompasses all frequency-dependent real and imaginary losses, including inductance, capacitance, conductor skin effect, and core losses in magnetic materials.